MS07 - CDEV-02
Mechanistic modeling from inter- to intra-cellular phenomena
Thursday, July 17 from 4:00pm - 5:40pm in Salon 15/16
SMB2025 Follow
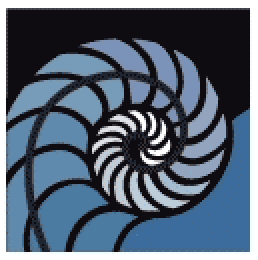
Organizers:
Andreas Buttenschoen (University of Massachusetts Amherst), Calina Copos (Northeastern University)
Description:
Processes such as the immune system response, cell division, developmental processes, and cell migration require the coordination of intra-cellular and inter-cellular processes from inside the cell to between cells, and across tissues. Defects manifest in pathological conditions such as cancer. Identifying key molecular players and their spatiotemporal dynamics requires a multiscale perspective, novel mathematical modeling, and data-driven inference. Ultimately, as a community we aim to identify the universal principles underpinning such cellular phenomena. In this minisymposium, we bring together emerging leaders and innovative early-career scientists who develop tools and models uncovering the mechanistic underpinnings of intra- and inter-cellular dynamics, and generate key insights using mathematical and statistical tools. The mini-symposium aims to bridge the gap between theoretical models and experimental data, fostering collaboration and innovation in the dynamic field of cellular research.
Room assignment: Salon 15/16
Jupiter Algorta
University of British Columbia"A Data-Driven Model of Polarity Reversal in Migrating Cells"
Mariya Savinov
New York University"Modeling mechanically driven tumor cluster coattraction on ECM"
Wei Wang
Johns Hopkins University"Statistics of fracture in collective cell migration"
Eric Cytrynbaum
University of British Columbia" A model for root zone regulation by brassinosteroid and CLASP"
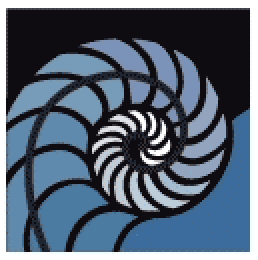